Abstract
Methionine (Met) is 1 of 8 essential amino acids in the human diet, and the only 1 that contains sulfur. It is the requisite substrate for homocysteine, cysteine, taurine, S-adenosyl methionine (SAMe) and polyamines. Methionine is also needed for cellular proliferation, specifically in protein and RNA synthesis. Metabolically, methionine acts as an anabolic stimulant. Restriction of methionine in the diet has been used in animal studies as a mimetic to caloric restriction with similar metabolic effects. Many of these effects, such as lower insulin-like growth factor (IGF), should induce favorable outcomes in patients with a history of cancer. This review explores the theory and evidence for a normal-calorie, methionine-restriction diet in the context of cancer care specifically.
Introduction
While dubbed essential, methionine is unique among the essential amino acids in that it is the only 1 whose long-term dietary deprivation is still compatible with life.1 Unlike the other essential amino acids, which must be ingested, methionine can be recycled by remethylation of homocysteine in normal cells. There are 2 possible remethylation pathways:
- Homocysteine can be converted to methionine by methionine synthase (MetS) in a methyl-tetrahydrofolate (folate) and methyl-cobalamin (B12) dependent reaction.
- Homocysteine can be remethylated by betaine-homocysteine methyltransferase (BHMT), a reaction requiring trimethylglycine (betaine) as the methyl donor.
As long as there is homocysteine, intact MetS and/or BHMT, and the requisite cofactors, the production of methionine is possible. This is true for all normally functioning cells. (Figure)
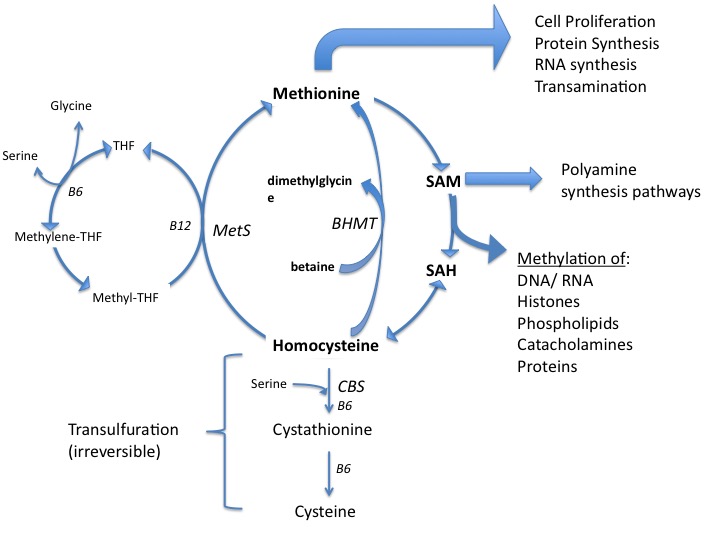
Figure. Methionine Utilization.
Most cancer cells do not have methionine cycle enzymes intact, though.2 As a result, they need to have methionine available for growth processes. This metabolic difference in methionine usage may allow for a targetable vulnerability in cancer cells. Noncancerous cells should be capable of surviving without methionine, while cancer cells would not. This has been demonstrated in animal models of various cancers.3,4
Methionine plays a role in many steps within the carcinogenic processes. Methionine is necessary for protein and RNA synthesis in all cells. Another role is as the substrate for S-adenosyl methionine (SAMe), which acts as an essential methyl donor for numerous molecules within cells, including methylation of DNA in the nucleus.5 Global hypomethylation (ie, reduced methylation that is genome-wide) is implicated in various cancers and results in chromosomal instability.6 Hypermethylation of promotor regions of tumor suppressor genes can effectively turn off the expression of those genes. These epigenetic changes of carcinogenesis are influenced by the microenvironment of the tumor, including the availability of methyl groups from the methionine/folate cycle.7,8,9,10 (This review will not include methionine’s role in carcinogenesis per se. Rather the effects of methionine, and its deprivation, on existing cancer are the scope of this review.)
Methionine is needed for the production of polyamines (putrescine, spermidine, spermine), which are involved in cellular division and are found in higher concentration in tumors.11 Polyamines decrease apoptosis, increase proliferation, and are involved in tumorigenesis.12 Their inhibition has been proposed as a means of reducing cancer risk.13 Preliminary trials limiting polyamines (through diet and gut ecology manipulation) in men with metastatic prostate cancer suggest such deprivation may significantly delay cancer-related mortality.14,15 While hypothetical, methionine restriction is expected to result in less polyamine production intracellularly.
A recent study of breast cancer cells found that methionine deprivation reduced growth of tumor-initiating cells (TICs) (ie, the stem cell niche).16 The authors propose that since methionine is required for protein synthesis, its deprivation serves as a block to necessary anabolic processes. The authors conclude, “Our results may also explain the anti-cancer health benefits of caloric restriction, intermittent fasting and the vegetarian diet, by systemically reducing protein synthesis in TICs.”
There is direct evidence that methionine restriction leads to selective death of cancer cells versus normal cells.
The effect of methionine restriction on whole organisms is such that proliferation and anabolic processes are lessened. In organisms from yeast to mammals, caloric restriction results in inhibition of insulin-like growth factor (IGF) signaling, activation of adenosine monophosphate activated kinase (AMPK), inhibition of mammalian target of rapamycin (mTOR), and reduction in mitochondrial oxidation.17,18,19 Disruption of such anabolic pathways also occurs with diets containing adequate calories that restrict methionine.20,21 IGF, AMPK, and mTOR are well-characterized pathways of cancer growth, making their disruption a desirable goal.22
In vitro, there is direct evidence that methionine restriction leads to selective death of cancer cells versus normal cells.23,24 Various cancer cell types cannot survive in media devoid of methionine even when homocysteine is present.25 Specifically, many cancer cells undergo cell cycle arrest and eventually apoptosis mediated by pro-apoptotic molecules such as Bcl-2.26 In addition, nutrient-sensing mechanisms within cells have been proposed as a means of organism control of weakened or defective cells. When certain nutrients, including methionine, are scarce, autophagy may ensue. 27
Ample animal evidence suggests that methionine restriction may affect the growth and/or metastasis of cancers. Methionine restriction in rodents has led to tumor regression in xenografts of prostate cancer.28,29 Sarcoma-bearing nude mice deprived of methionine showed longer survival and regression of tumors.28 Rodents with implanted brain tumor xenografts achieved stability or regression with methionine restriction. Methionine restriction has also lessened metastatic spread of sarcoma tumors in mice.30 Lastly, methionine restriction has demonstrated a potentiation of chemotherapy in mice with implanted brain tumors.31
Ongoing research continues to define effects of methionine deprivation on cancer cells. Cellular disruption of methylation, protein synthesis and intracellular signaling pathways normally stimulated by growth factors such as IGF and mTOR have all been demonstrated.32 The net effect is a reduction of proliferation. While this data is fairly consistent in cell culture and animal models, the translation to humans is much more complicated. Methionine is an essential amino acid. As such, ramifications of its restriction must be weighed against any benefits.
Methionine Restriction Trials
A handful of phase I studies have assessed the safety and feasibility of iso-caloric diets with methionine restriction in patients with cancer. While the diets have resulted in weight loss, methionine restriction has been generally well tolerated and has not resulted in any nutritional deficiencies. Long-term use and follow up of methionine-restricted diets in patients with cancer or a history of cancer have not yet been the subject of clinical trials.
Methionine restriction may complement chemotherapies targeting the methionine/folate cycle specifically. In 1995, 14 patients awaiting surgical resection of gastric cancer were given continuous infusion of 5-fluorouracil (5-FU) for 1 week before surgery. All participants also received total parenteral nutrition (TPN) for that week, with half (n=7) given TPN without sulfur-containing amino acids (methionine and cysteine).33 After resection, only those receiving sulfur-free TPN had histologic cancer cell degeneration and better thymidylate synthase inhibition (ie, the mechanism of 5-FU). This trial suggests possible synergy with 5-FU and is the only trial in which TPN was used, providing complete control over nutrient intake and the ability to achieve no methionine ingestion at all.
In 2002, Epner and colleagues published a phase I study of 8 people with metastatic cancer and concluded that methionine restricted diets are feasible and well tolerated over the short/medium term. Restriction entailed prescribed diets using a commercial methionine-free amino acid blend (Hominex-2, Abbott Labs) as the primary protein source. The diet contained 0.6-0.8 g of total protein, 25-35 kcal, and 2 mg of methionine per kilogram of body weight per day. Participants remained on the experimental diet for 8-39 weeks (mean=17.3 weeks). Circulating methionine was reduced an average of 58% (from 21.6 +/- 7.3 µm to 9 +/- 4 µm) within 2 weeks of start of treatment. Homocysteine was lowered only slightly. Serum albumin levels remained stable or increased. Daily protein targets were met and overall caloric energy intake increased. The only side effect was weight loss of approximately 0.5% of BMI weekly.34
In a follow-up publication, Epner commented on this phase I trial stating that 1 patient with hormone-independent prostate cancer experienced a 25% reduction in PSA after 12 weeks on the trial.35 Another patient with progressive renal cell carcinoma had a radiographic objective response to treatment. While these results were incidental to the design of this phase I trial, they are intriguing.
A single day of a methionine-restricted diet appears to lower circulating levels of methionine dramatically. A 2008 phase I study looked at methionine restriction alongside the chemotherapy drug cystemustine in patients with metastatic melanoma or glioma.36 The study assessed circulating methionine after 1, 2, 3, and 4 days of methionine-restricted diet. After day 1, study participants experienced an average 41% reduction in circulating methionine without appreciable further lessening in the ensuing days.
Based on the phase I finding, the same researchers designed a phase II trial to assess the safety and efficacy (toxicity and nutritional status) of cystemustine with 1 day of methionine restriction before each biweekly treatment.37 Twenty patients with metastatic melanoma and 2 with glioma received an average of 4 cycles of cystemustine. While toxicity of treatment did not change and nutritional status was preserved, the outcomes were not impressive. The patients experienced no improvement in disease-free survival (median=1.8 months) nor overall survival (median=4.6 months). The authors point out that the patients recruited had very poor prognosis and/or were heavily pretreated. One patient had 27 months of stable disease.
Compliance with a methionine restriction diet, even short term, may be a challenge. In a publication in 2010, 11 patients undergoing combination chemotherapy FOLFOX (5-FU, leucovorin, oxaliplatin) over a 48-hour period underwent methionine deprivation diet for 3 days around treatment.38 Methionine levels fell an average 58% by day 1. Only 4 patients were evaluable at the end of treatment due to difficulty in adhering to the methionine restriction diet. Of these 4, 3 had partial responses to FOLFOX and 1 had stable disease.
These phase I/II trials demonstrate that methionine restriction is feasible short term. The 2010 study suggests instituting a low-methionine diet may be difficult for patients, at least if done without the use of a supplemental low-methionine meal-replacement product. As several of the studies demonstrated, weight loss is also very likely, even if the diet contains adequate calories. These are very practical hurdles that may limit the utility of methionine restriction diets clinically.
Methioninase Agents in Development
Given the compliance challenges, an ideal solution would be to remove methionine from cells, rendering them deficient in methionine without requiring dietary deprivation. Methioninase derivatives using recombinant DNA (rDNA) derived from bacteria are in development.39 Because of the concerns about the antigenicity of rDNA methioninase directly infused into the bloodstream, less antigenic conjugates and liposomes are also being researched.40,41 Overall, methioninase agents are promising in their low toxicity and serve as another means of deducing response to a low-methionine environment.
In 1996, Hoffman and colleagues published the first phase I trial of intravenous methioninase derived from Pseudomonas putida in 3 patients with metastatic breast cancer.42 Each woman received a different dose: The first 2 received 5,000 units or 10,000 units over 2 hours, and the third woman received 20,000 units over 10 hours. Depletion of serum methionine began within 30 minutes of infusion. Serum methionine was reduced by 35% and 19% in the first 2 patients and reached a 200-fold reduction (from 23.1 µm to 0.1 µm) in the third patient. None of the patients experienced toxicity. This pilot phase I trial established safety and tolerability. Disease outcome measures were not reported.
A year later, Hoffman and colleagues again published a study on direct infusion of the same rDNA methioninase in patients with breast, lung, or renal cancer or lymphoma. They confirmed that a 6-24 hour infusion of doses ranging from 5,000-20,000 units had no toxicity in late-stage cancer patients.43 The reduction of methionine was as low as 0.1% of baseline. In a 2015 paper looking back at the “40 year odyssey” of methionine research, Hoffman proposed the most strategic use of methioninases might be in conjunction with certain chemotherapeutics.44 Methioninases arrest cancer cells in the S/G2 phase of the cell cycle. Combining chemotherapeutic agents that also target this stage may result in a synergistic effect. This sequential use of using methioninases to arrest the cancer cells and giving a cell cycle–specific cytoxic agent has demonstrated some efficacy in vitro, particularly with paclitaxel.45, 46,47
There is a caveat to the cell cycle arrest from rDNA methioninase. Some in vitro research indicates that the phase of the cell cycle that cells are arrested in is different with methionine deprivation versus methioninase application. Methionine-deprived prostate cancer cells (PC-3) were arrested in the G2/M phase, while methioninase arrested the cell cycle at the S/G2 phase.48 This differentiation may influence whether there is synergy or antagonism with a given chemotherapeutic.
Discussion
Caloric restriction results in increased longevity across various species, including flies, fish, and dogs.49,50,51 Within oncology, caloric restriction has demonstrated decreased side effects of chemotherapy treatments.52,53 Excess energy balance, such as a diabetic state and obesity, are implicated in poorer outcomes in those with a history of cancer.54,55,56 Caloric restriction is a feasible option for those who are overweight or obese, at least until normal weight is achieved. However, long-term caloric restriction may result in nutrient deficiencies that influence bone health, immune function, or sarcopenia.57 Methionine restriction may offer a means of achieving similar physiological effects with less severe caloric restriction and higher nutrient intake.
Methionine occurs in all proteins in nature, to some extent. Overall, methionine is generally highest in fish, beef, dairy, eggs, nuts, seeds, and grains, respectively, when looked at on a mg per serving basis.58 Vegetables and fruits have the lowest content of methionine due to lower protein amounts. As a percentage of protein content, nuts tend to have the highest percent concentration (mg methionine/gram protein); however, since animal sources have more protein per serving, the content of methionine is higher for practical purposes. Thus, a vegan diet is fairly low in methionine. It does not, however, reach methionine restriction levels used in the studies mentioned above (2 mg methionine/kg body weight/day).
Whether humans are able to arrest cancerous growth with methionine restriction, as established in animals, is not known. There are no prospective trials of methionine restriction designed to measure outcome in cancer survivors. While speculative, methionine restriction does result in effects expected to have physiological benefit for those with a history of cancer. These effects include lessening of IGF-1 production, improved insulin sensitivity of normal cells,59 increased fat oxidation,60 and lessening of inflammation overall.61
A low-methionine therapeutic diet has been established for people with inborn errors of metabolism of the methionine cycle. The most common of these errors is cystathionine beta synthase (CBS) deficiency,62 the enzyme needed to commit homocysteine to the transulfuration pathway toward cysteine. The effect of a defect in CBS is a backup of methionine and homocysteine in the blood. Therapeutically, those affected by CBS must be put on a low-protein/low-methionine diet. Since all food in nature that contains protein has methionine, specially formulated medical food products have been created with only trace amounts of methionine to serve as the primary protein source for those with CBS.63 These medical foods were used to achieve the ultra-low levels of methionine in several of the phase I studies discussed above.
The requisite amount of methionine for human health appears to be approximately 13 mg/kg body weight/day.64 Many of the methionine-deficient diets used in the clinical trials contained 2 mg/kg body weight/day of methionine and 0.6-0.8 g/kg body weight/day of overall protein in the diet. Long-term effects of this restriction have not been addressed. It is presumed that 0.5% of BMI loss per week (as reported in phase I trials) is likely. Methionine restriction appears to effectively lower visceral adiposity,65 which is desirable in those with cancer, but muscle loss can be a risk as well. Muscle degradation limit immune function, as the body will prioritize muscle preservation over immunity at some point in an ongoing sarcopenic state.66,67
While calorie- or methionine-restrictive diets appear to increase longevity across species, at least 1 rodent study suggests that reproductive function is best with a more protein-rich diet.68 Looking at ratios of macronutrients, longevity in male and female rats was optimal at protein:carbohydrate (P:C) ratios of 1:13 in male rats and 1:11 in female rats. Reproductive health was optimal at 1:1 ratio for both genders. The practical impact of this, even if it holds true in humans, is limited only to those considering conception. This is pertinent only to a relative small number of patients with cancer.
The rodent studies are consistent regarding increased longevity. However, human data is more complicated regarding protein intake specifically. An intriguing study published in 2014 suggested stratification of the effects of protein intake by both age and food source. Dietary intake of 6,381 adults ages 50 and over (average age 65) from NHANES III was divided into high protein (20% or more of calories from proteins), moderate protein (10-19%) or low protein groups (<10% of calories from protein).69 High protein intake was associated with increased diabetes mortality across all ages. In those 50-65 years old, high protein intake had a 74% (HR: 1.74; 95% CI: 1.02–2.97) increase in all-cause mortality and a 4-fold (HR: 4.33; 95% CI: 1.96-9.56) increase in cancer death risk during the following 18 years. When the percent of calories from protein was controlled for by type of protein (animal or plant), only animal protein was associated with the apparent detrimental effects of a high-protein diet. Moderate protein consumers (age 50-65) had a 3-fold increase risk of cancer (HR: 3.06; 95% CI: 1.49-6.25). These effects were slightly mitigated in those who consumed plant protein over animal protein. Interestingly, high protein intake for those over 65 years old was associated with reduced cancer and overall mortality. The authors conclude that the detrimental effects of muscle wasting through low protein in the elderly may outweigh other metabolic effects. The concept of higher protein needs (1.0-1.2g/kg body weight/day) in the elderly (>65 years old) is supported by a 2014 consensus report of the European Society for Clinical Nutrition and Metabolism.70
Sufficient research exists regarding bone health in postmenopausal women to remain cautious of protein restriction in this population as well. The anabolic pathways involved in bone growth include mTOR and IGF-1; stimulation of these pathways in bone is desirable. This is precisely the opposite of the mechanisms behind longevity and cancer growth interference. In addition, muscle mass is more apt to degrade with age; preservation is stimulatory for bone growth and improves balance, leading to fewer falls. Given the clinical implications of protein deprivation, caution should be used in elderly women. In general, the protein recommendation for postmenopausal women with osteoporosis/osteopenia is 1.0-1.2 g/kg body weight/day.71
These considerations must be weighed against the clinical utility of anything at our disposal that can slow, stabilize, or even regress cancers in our patients with poor prognoses. Diets high in vegetables/legumes/grains and fruits (>two-thirds of the diet being plant-based) are well evidenced as the best diet for prevention of cancer.72,73 To what extent a high plant-based diet influences outcomes once cancer is present is a subject of ongoing debate, with a less obvious conclusion.74, 75,76,77,78
It should be noted that there is some evidence that ketogenic diets may improve outcome in metastatic cancer patients.79 A true ketogenic diet requires restriction not only of carbohydrates, but of protein as well, as both are capable of increasing circulating glucose. The mechanism behind any benefit to the ketogenic diet in lessening cancer progression may be due to inadvertent methionine restriction.80 Ongoing research should clarify this; high-fat diets using adequate protein (Atkins diet) and restricting carbohydrates only have been studied.81
There is no outcome data on methionine restriction diets specifically used as an intervention in patients with cancers. For practical purposes, vegan diets, with their lower levels of methionine, are a feasible option. Vegans can meet their protein needs while minimizing methionine when compared to an omnivore diet. In addition, large quantities of vegetables, fruits, and legumes are required to meet caloric needs. As long as the diet relies on whole foods, a vegan diet can increase nutrient intake, minimize methionine, and displace less nourishing foods.82
In practice, we must meet each patient where he/she is. The patient’s psychology around food, current body composition, current state of health/disease, finances, time available to prepare food, and beliefs about food are all considerations. Ultimately, creating excess stress through dietary goals that are not attainable may be more harmful than finding a diet that can be both enjoyable and advantageous to health and longevity.83
Conclusion
Methionine restriction diets have been used as a substitute for caloric restriction in animal models and result in similar physiological changes. Given the methionine dependence of various cancer cells, methionine-restricted diets are an intriguing option for those with a history of cancer. While long-term caloric restriction poses the risk of decreasing weight to a detrimental extent,84 methionine restriction without calorie restriction may confer the same benefits while lessening this risk.85 Much more research needs to be done to clarify whether there is indeed benefit to methionine restriction, and if so, which cancer types would be helped and how much restriction is sufficient.